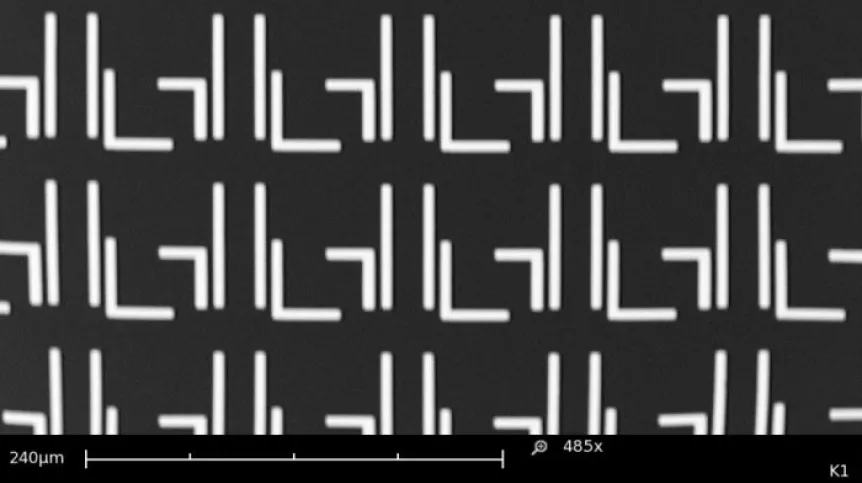
Researchers at the Military University of Technology are developing tunable hybrid metamaterials that open up the possibility of designing active microcircuits with the ability to switch, modulate, slow down and accelerate terahertz waves. They can be a platform for building multifunctional photonic devices such as biochemical sensors, absorbers, filters, non-linear switches whose properties can be actively modulated.
Dr. Rafał Kowerdziej from the Faculty of New Technologies and Chemistry of the Military University of Technology, winner of the SONATA BIS competition organized by the Polish National Science Centre, talks about the project. The interdisciplinary team to be formed as part of the project will simulate, characterize and optimise the tunable properties of the so-called fast light (FL) and slow light (SL) effects, occurring in ultrafast terahertz metamaterials, based on the plasmon-induced transparency effect.
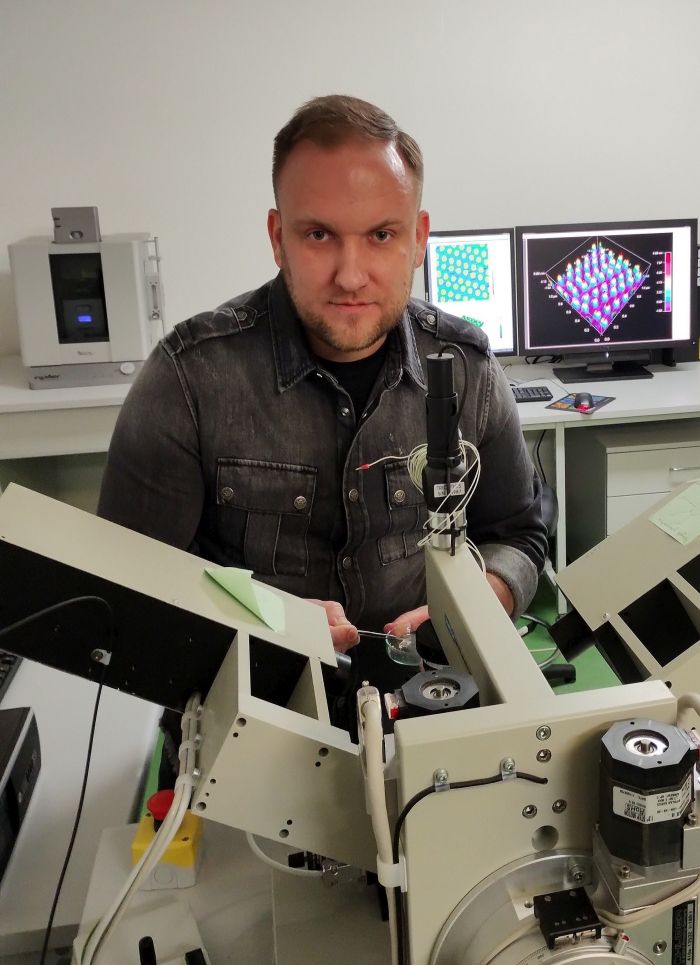
PAP: How can you explain terahertz metamaterials in a simple way?
Dr. Rafał Kowerdziej: The term metamaterial is now widely recognized as synonymous with futuristic material. Note that the Greek word 'meta' means over, beyond. Thus, metamaterials can be described as materials with properties beyond or exceeding those of the ordinary or 'natural' materials, from which they are built.
From the physical point of view, we can say that electromagnetic metamaterials are microscopic composite structures produced using modern technologies (such as electrolithography) that do not occur in nature - most often of the metal-dielectric type (although dielectric metastructures are gaining popularity - made of dielectric materials with high refractive index, most often amorphous silicon), which interact resonantly with the electromagnetic field.
The metastructure is built on the basis of the so-called elementary cells, which are periodically distributed metallic elements called resonators (made of gold, silver or aluminium) deployed on dielectric substrates (such as quartz glass). We must emphasize here that the dimensions of the elementary cells should be several times smaller than the length of the wave propagating through the structure.
The terahertz metamaterials studied in our project are metal-dielectric plasmonic materials with resonance frequencies in the 0.3-3 THz (terahertz) range. In the case of metastructures in the THz range, the dimensions of elementary cells range from several dozen to several hundred micrometers, and a single metasurface may consist of several thousand elementary cells. Terahertz metamaterials will be made of precious metals (mainly gold and silver) on quartz substrates. Their physical parameters will be determined and then optimised in a series of electromagnetic simulation processes.
PAP: Now that we know more about terahertz metamaterials, the next step to understanding the idea of the project is to learn about the plasmon-induced transparency effect...
R.K.: Metamaterials based on the plasmon-induced transparency effect are usually made of elementary cells containing at least two metallic elements. I will try to explain this phenomenon using the simplest example. Suppose that two metallic rods are placed in one elementary cell of a metamaterial. If we properly model the geometric dimensions of these rods and the distance between them, and then illuminate the structure with terahertz radiation, we will observe the occurrence of two resonant modes, the light mode and dark mode - if the rods are perpendicular to each other, or two light modes - if the rods are parallel to each other and at the same time the electric field of the electromagnetic wave is parallel to the long axes of these rods. Both of these modes are somehow assigned to the metallic elements that make up the metamaterial.
What are these mods? If we arrange the rods within the unit cell so that they are perpendicular to each other, then the light mode will be excited directly by the electric field of the electromagnetic wave in the rod, which is parallel to the electric field strength vector of the electromagnetic wave, which results in a strong excitation of surface plasmons at the boundary of the metallic rod forming the metastructure and the dielectric substrate. As a result of the action of the electric field, the charge in the metallic rod shifts, as a result of which it becomes a de facto electric dipole. Note that the resonance curve of the light mode is deep and narrow, which means that it has a high Q factor.
In contrast to the light mode, the dark mode - assigned in this case to the rod whose long axis is perpendicular to the electric field strength vector - cannot be excited by the electric field of an electromagnetic wave, therefore the resonance curve of this mode is wide, i.e. it has a low quality factor. As such, these two mods have extremely different quality factors. The plasmon-induced transparency effect in metamaterials is the result of the interference (overlap) of these resonance modes.
In metamaterials based on the plasmon-induced transparency effect, it is crucial to properly design the metastructure, i.e. carefully select and optimise geometric dimensions, mutual arrangement of metallic elements within the cell and appropriately model the distance between metallic elements - so that the two modes can couple. The plasmon-induced transparency effect manifests itself in the appearance of a narrow window of transparency (a peak in the form of high transmission) in a wide absorption spectrum.
PAP: The consequence of the plasmon-induced transparency effect are the so-called fast and slow light effects. What are they exactly?
R.K.: Both of these effects are related to the group velocity of an electromagnetic wave that propagates in a dispersive medium, i.e. one whose index of refraction (n) depends on the frequency of the wave (ɷ). Of course, in our case, this medium is a metamaterial.
In a material with a frequency-dependent refractive index, each frequency propagates with a different phase velocity, thus modifying the nature of the interference. If n(ω) changes linearly with wave frequency ω, the modified interference effect is the pulse peak shift over time, but with the pulse shape unchanged.
The fact that the pulse is time-shifted means that it is moving at a speed different from the phase velocity. We refer to this 'new' velocity as the group velocity. Comparing the relationships describing the phase and group velocities, we can see that the relationship describing the group velocities additionally includes a coefficient describing the dispersion dn/dω of the refractive index.
To obtain the 'fast light' effect, it is necessary to design a metamaterial for which dn/dω is as high as possible and negative. In the case of metamaterials based on the plasmon-induced transparency effect, this is achieved by exploiting the strong change in refractive index that occurs near the resonance of the metamaterial.
What is perhaps the most significant thing in recent research on fast light is not the existence of an effect, but rather the realization that certain pulse shapes can propagate in a highly dispersive medium with negligible pulse distortion. In order to prevent pulse distortion, higher order dispersion - resulting from the non-linear dependence of the refractive index on the frequency of the wave - must be minimized.
In other words, it (the 'fast light' effect) boils down to the condition that the group velocity of the electromagnetic wave must be negative. In turn, the slow light phenomenon/effect can be defined as the propagation of light pulses or other light modulation modes at a relatively low group velocity. To achieve this, we need to model the metastructure in such a way that the refractive index dispersion (dn/dω) is as high as possible and positive.
PAP: What is the purpose of your team's research?
R.K.: As part of the proposed project, we intend to establish an interdisciplinary research team to simulate, characterize and optimise the tunable properties of the PIT, SL and FL effects occurring in hybrid terahertz metamaterials. Tunable parameters of metamaterials will be obtained by using newly developed liquid crystal materials, vanadium dioxide (VO2) and molybdenum disulfide (MoS2), for the construction of transducers.
The implementation of hybrid metamaterials will allow us to tune PIT, SL and FL effects electrically, thermally and through photoexcitation, and obtain picosecond switching times. Importantly, the proposed methodology enables tuning of the above-mentioned properties of metamaterials in an ultra-fast, low-energy, continuous and reversible manner (as opposed to most existing methods, which only allow resonance switching between on and off states).
Tunable hybrid metamaterials open up possibilities for designing active microcircuits with the ability to switch, modulate and 'slow down and speed up terahertz waves'. They can be a platform for the construction of multifunctional photonic devices, such as biochemical sensors, absorbers, filters, non-linear switches whose properties can be actively modulated, and thus cannot be obtained with the technologies used so far.
The final result of the research/project will be tunable metamaterial transducers based on the plasmon-induced transparency effect and the technology for their implementation. In addition, we will get an answer to the question of what limit and optimal switching/tuning times of the PIT, SL and FL effects can be obtained by using liquid crystals, VO2 and MoS2 for the construction of transducers.
PAP: Thank you for the interview.
SEM image of a terahertz metamaterial based on plasmon-induced transparency effect. Credit: Dr. Rafał Kowerdziej
The project 'Tunable and ultrafast terahertz hybrid metamaterials based on the plasmon-induced transparency effect' received funding in the amount of PLN 1,676,890. As part of the grant, Dr. Rafał Kowerdziej's research team will collaborate with Professor Roberto Caputo's team from the University of Calabria.
PAP - Science in Poland, Magdalena Barcz
bar/ zan/ kap/
tr. RL