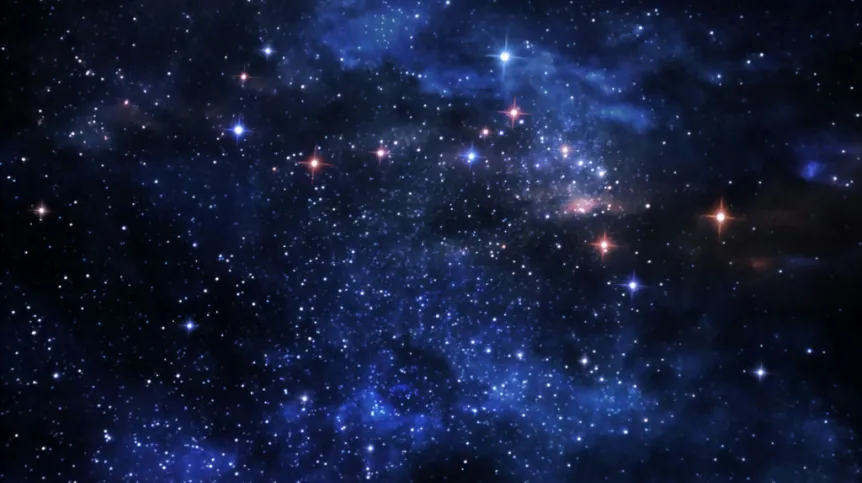
Scientists have tested Einstein's theory of General Relativity (GR) for deviations with the LIGO/Virgo detector network.
After implementing nine different methods to verify the consistency of Einstein's theory with the observational data, no discrepancies were found. Polish scientists from the Polgraw group, including scientists from the National Centre for Nuclear Research, were involved in the project.
The National Centre for Nuclear Research (NCBJ, Narodowe Centrum Badań Jądrowych) informed about their research in a press release prepared by Małgorzata Bankowicz and Adam Zadrożny.
General Relativity, proposed over 100 years ago by Albert Einstein, is now the widely accepted theory of gravity. The theory correctly describes the known astronomical phenomena driven by gravity, and serves as the basis for the construction of cosmological scenarios. As research and observations progress and larger, more accurate and well-ordered data sets are collected, the area of available phenomena continues to expand.
Professor Marek Biesiada from the NCBJ Astrophysics Division said: “In science, we do not treat any theory as a dogma. That is why we test theories, constantly checking their predictions. So far, GR has been confirmed by very precise observations in the Solar System and in binary pulsar systems. Gravitational waves emitted by merging black holes provide another opportunity to test General Relativity. It is a regime of highly curved spacetime, previously poorly available for testing.”
According to a press release from the NCBJ, there are at least two reasons to check whether GR requires modification or replacement with a new theory. The first are the cosmological problems known as dark matter and dark energy. The problem with dark matter is that galaxies and their clusters attract more strongly than they should if all matter we know is taken into account. The problem with dark energy is that the Universe is accelerating its expansion, rather than slowing it down as GR would predict. While the working names dark matter and dark energy suggest a response in the form of unknown material components, the possibility remains that GR requires modification. The second premise is the necessity (resulting from GR) of singularities, or areas where the stories of all particles and photons end. This problem seems to be related to the quantum theory of gravity, which has not yet been created in a form satisfactory to all. Here, too, the gravitational waves emitted by merging black holes can provide clues.
The LIGO and Virgo Scientific Collaborations published this week a summary of the analysis of the data they had collected in terms of their consistency with the GR predictions. The analyses were presented in 9 main groups constituting tests of the theory, the NCBJ reports.
The first test concerned the consistency of the recorded base signal (noise) with the detector noise known from laboratory tests. Based on GR, we know what the signal from two compact objects should look like in gravitational wave detectors.
The press release said: “But what we use to describe a signal is a theory, like all science, it is an approximation, the best one we have, describing the world until we find a better one. If GR did not describe such signals well enough, we would have theoretical prediction plus an additional component derived from the effects not taken into account. In order to see if such an additional component was present, it was necessary to check whether, after subtracting the predicted GR waveform, the residual would be consistent with the detector noise. The test confirmed the validity of GR.”
Waveform consistency before and after the merging of two objects was also tested. According to the NCBJ: “The sources of gravitational waves that we observe are the systems of two neutron stars; two black holes; or black hole - neutron star. The merger event of these objects takes place in 3 main phases: the moment just before the collision, the moment of merger and the stabilization phase. GR predicts that the pre- and post-merger phases should generate similar waves.
The GR predictions are consistent with the observations for the analysed sample. The next two tests concerned the behaviour of objects in the first phase of merging, the slow inspiral of the two bodies toward each other.
The inspiral of two dense objects, such as black holes and neutron stars, approaching each other due to the loss of energy emitted in the form of gravitational waves, can be approximated by a weak-field, slow-motion, known as the post-Newtonian approximation. This approach is described by several parameters, the determination of which on this basis can be compared with the parameters obtained by GR. The latest observations, combined with the previous ones, provide state-of-the-art bounds on the deviation coefficients. These results are statistically consistent with the GR predictions.
The inspiral phase of the binary also allows us to test whether the signal is consistent with the coalescence of two spinning (Kerr) black holes in GR. If one of the compact objects is spinning, the resulting object will be flattened at the poles and bulging at the equator. Scientists are able to extract this information from observational data, which allows to determine that sources of gravitational waves are not any exotic objects, not predicted by GR.
A similar approach was used to determine the parameters of the event during and after the merger of the objects. The duration of the merger and stabilization of the new object is much shorter than the inspiral, so the observed signal is much stronger than the visible noise. The values of parameters estimated on this basis are statistically consistent with the GR predictions.
Another test concerns gravitational wave propagation, the NCBJ reports. According to the GR predictions, gravitational waves propagate non-dispersively, which means that the speed of their propagation does not depend on their frequency. GR can be modified in such a way that this property is not retained. In such a situation, waves originating directly from a merger of objects with a higher frequency would reach the observer faster than waves with a lower frequency originating from the inspiral phase. No evidence of gravitational wave dispersion was found, which is consistent the predictions of GR, the press release reads.
The lack of the observed dispersion makes it possible to limit the models of particle physics that assume that gravitons, the particles responsible for the gravitational interactions, are assumed to have a tiny (but unknown) mass (the heavy graviton model). In GR, gravitons should be massless and travel at the speed of light. However, heavy graviton models predict the existence of dispersion to some extent, so observations allow to obtain a bound on the mass of gravitons. In these tests, the bound obtained for the graviton mass (if any) was determined to be below 1.3 times 10-23 eV/c2.
The eighth test concerns the polarization of gravitational waves. In GR, gravitational waves can only have two states of polarization: plus and cross. A more general theory of gravity can have up to six different polarization modes. The data from both LIGO and Virgo detectors were analysed for non-GR polarization content. The tests did not show any states of polarization other than those predicted by GR.
There are theoretical alternatives to black holes. These objects are referred to as black hole mimickers, because they can mimic the properties of black holes but are not GR black holes. One of the most distinguishing properties of a black hole is the presence of an event horizon, a surface from which nothing escapes, even light. In black hole mimickers, the equivalent of an event horizon may be a surface which may be partly or fully reflecting. This would cause exhoes in the third phase of merger. The analyses did not show the existence of any echoes, which is consistent the predictions of GR.
Dr. Adam Zadrożny from the NCBJ Astrophysics Division, and a member of the Polish research group Polgraw, said: “These are not all tests that the theory of gravity can be subjected to thanks to the study of gravitational waves.
“A very interesting example was the measurement of the Hubble constant for the observation of gravitational waves GW170817 and the optical flare AT 2017gfo, which were the result of the same event. This was reported in 2017 in Nature (vol. 551, p. 85–88). The measurement of the Hubble constant using data from gravitational wave detectors was consistent with results obtained by other methods. It is also worth adding that in their work in the 1980s, Professor Andrzej Królak (Institute of Mathematics PAS and NCBJ) together with Professor Bernard F. Schutz (Cardiff University) provided the basis for many methods of data analysis from interferometric detectors such as LIGO and Virgo.”
PAP - Science in Poland
ekr/ kap/
tr. RL